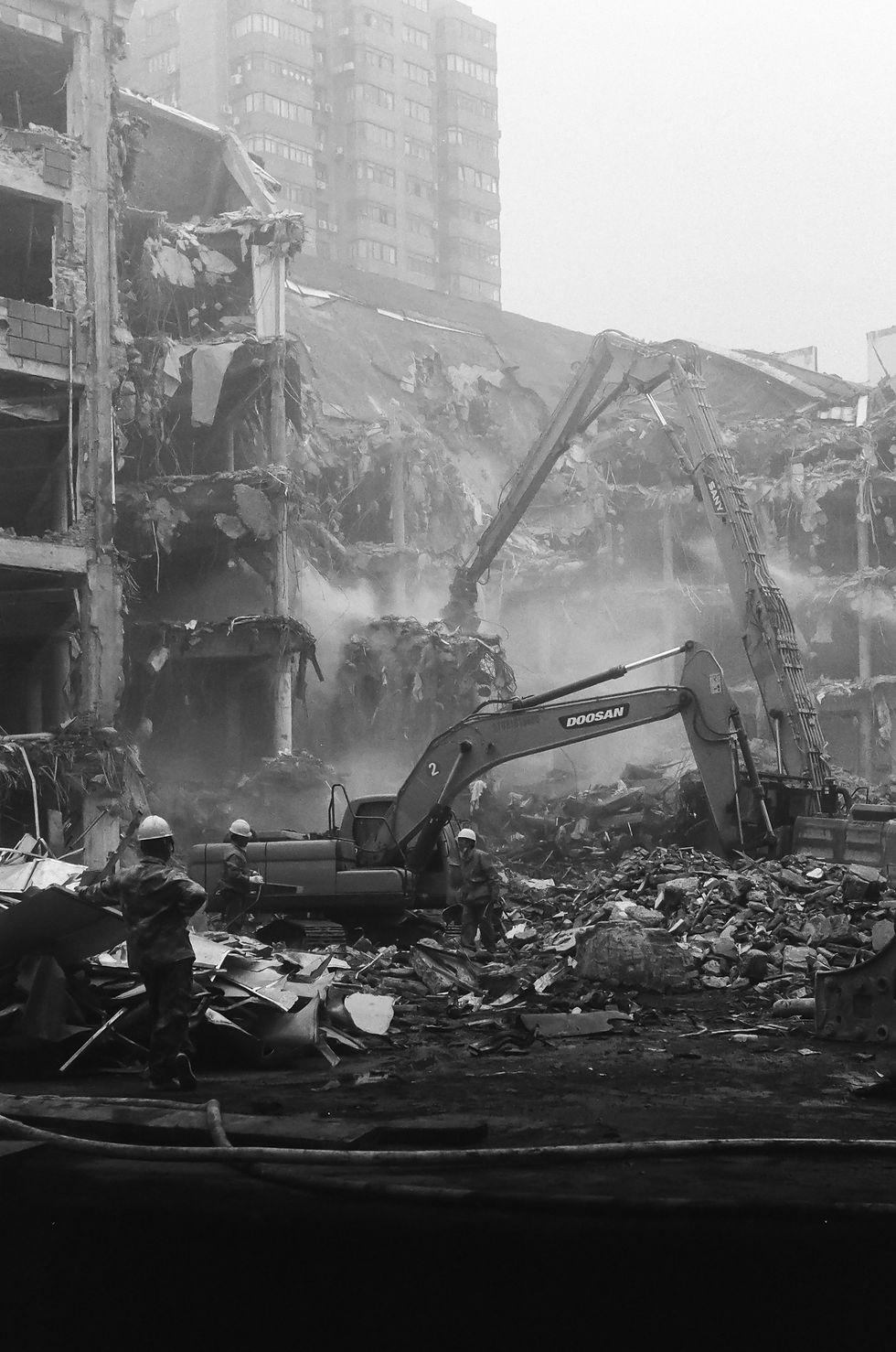
Introduction
As seismic activity continues to rise globally, the demand for more resilient infrastructure is becoming increasingly critical. Traditional materials like concrete and steel have been the backbone of structural design, but their limitations, particularly in high-seismic regions, are well-documented (Fig. 1).
In response, the field of seismic-resistant structures is evolving, with innovative materials offering enhanced safety and durability. These fibre-reinforced advanced materials are specifically engineered to absorb and dissipate energy during earthquakes, minimizing structural damage and saving lives.
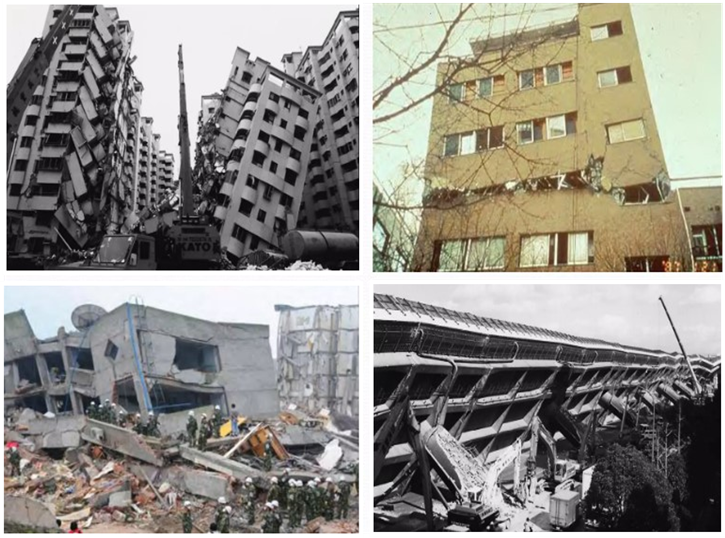
While current seismic design codes, such as Eurocodes [1], AASHTO [2], and Indian standards [3], provide robust guidelines, they also highlight the need for better-performing materials in response to extreme loads. This article delves into the materials that have the capability to transform the landscape of seismic-resistant construction (Fig. 2), ensuring that our infrastructure is more prepared than ever to face the unpredictability of high magnitude earthquakes. See material list:
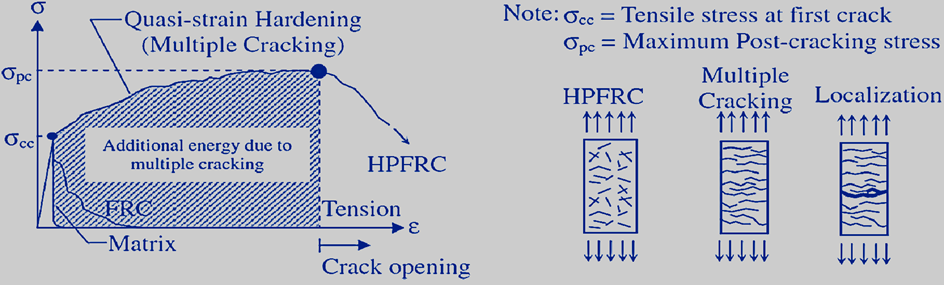
Shifting the Paradigm in Structural Design
Traditional materials like concrete, while durable, have inherent limitations when exposed to seismic forces. Under extreme lateral loads, these materials may exhibit brittle failure, compromising structural integrity (Fig. 1) [4]. This has led to the development of new materials designed to withstand the unpredictable nature of seismic events.
One such material is HPFRC, which offers improved ductility and crack resistance compared to standard concrete with better aesthetics (Fig. 3a). Another breakthrough is ECC, known for its strain-hardening capabilities and ability to endure tensile stress without breaking (Fig. 3b and Table 1). Additionally, 3DCP allows for the precise placement of these advanced materials (Fig. 3c), optimizing their performance in seismic zones.
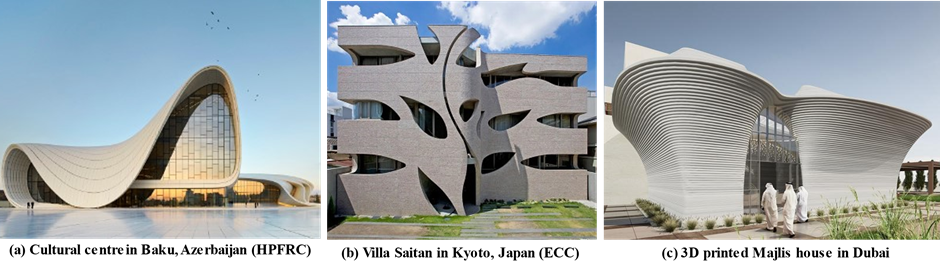
Seismic design codes such as Eurocodes (EN 1998-1), AASHTO (LRFD Bridge Design Specifications), and Indian Standards (IS 1893:2016) underline the importance of material ductility and energy absorption in seismic designs.
For instance, Eurocode 8 specifies the use of materials with enhanced energy dissipation and deformation capabilities. These codes reinforce the necessity of adopting materials like HPFRC and ECC, which provide higher performance under seismic conditions, as well as construction innovations like 3DCP, which facilitate the use of these materials in complex designs.[1] [2]
Material Innovations in Seismic Resistance
New materials for seismic-resistant structures are developed with specific properties that make them better suited to withstand seismic loads. HPFRC, ECC, and 3DCP stand out for their ductility, meaning they can undergo significant deformation without fracturing (Table 1).
This is a key feature when considering that seismic forces can cause rapid, unpredictable movements. These innovative materials are designed with strain-hardening properties, meaning that after initial cracking, they can continue to deform under stress, increasing their load-bearing capacity.
Material | Fiber types | Compressive strength (MPa) | Tensile strength (MPa) | Tensile strain capacity (%) | Reference |
ECC | PVA, PE, or PP fibers | 20–95 | 2–6 | 1–8 | Yi (2022) [5] |
HPFRC | Hybrid (PVA and steel) fibers | 40–45 | 1–3 | 0.1–0.5 | |
3DCP | Steel fibers | 20–40 | 1–4 | 1-3.5 |
Table 1. Summary of major high-performance materials
Another important property is self-healing, which is a feature of some modern cementitious composites such as ECC (Fig. 4) [8]. This means that small cracks, which typically propagate in traditional concrete under stress can close naturally over time, preserving the material’s integrity.

These innovative materials are also notable for their superior energy absorption, which allows them to dissipate seismic forces before they cause major structural damage. For example, 3D printed lattice structures using advanced cementitious composites can absorb and redistribute more seismic energy across the structure, reducing local stress concentrations compared to conventional concrete (Fig. 5).
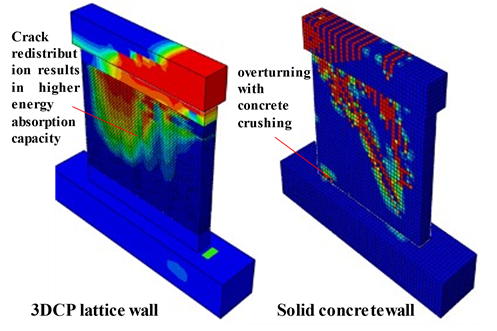
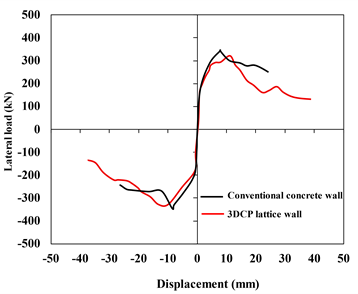
In addition to these innovations, the incorporation of steel fibers into concrete matrices has significantly improved the tensile strength and post-cracking behavior of seismic-resistant walls and columns. This advancement offers a more comprehensive approach to ensuring that critical infrastructure can survive intense seismic activity with minimal damage.
Also Read:
Case Studies of Innovative Material Applications
Several notable case studies illustrate the real-world application of these new materials in seismic-resistant structures:
1. Rokko Island in Japan: This artificial island, constructed using ECC for its earthquake-resistant seawalls, has proven to be highly resilient. During a recent seismic event, the ECC structures exhibited minimal damage, while nearby conventional concrete structures suffered significant cracks and deterioration.
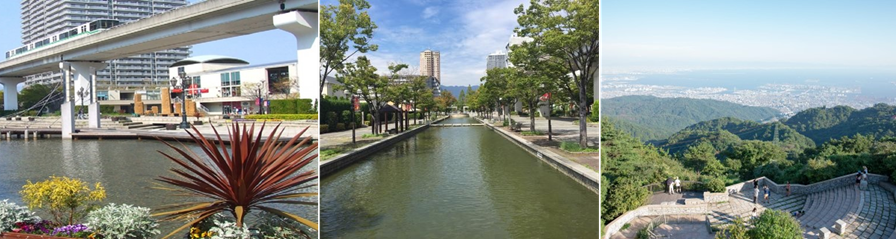
2. Mexico City’s Torre Reforma: As one of the tallest buildings in a highly seismic area, the Torre Reforma incorporates HPFRC in its core structure. This allowed for greater flexibility and energy dissipation during the 2017 earthquake of 7.1 magnitude,[3] [4] [SF5] outperforming neighboring buildings constructed with traditional concrete.
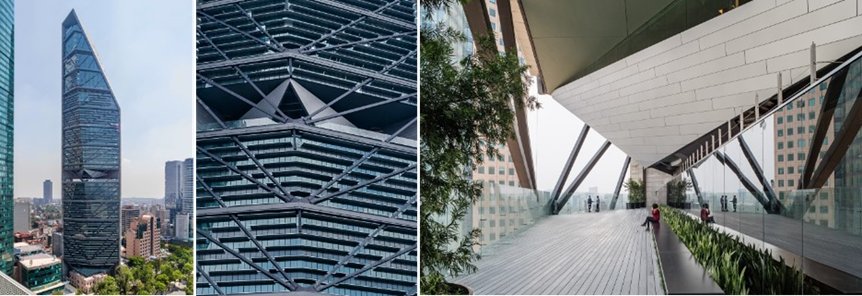
3. The 3D printed seismic house in Guatemala: Using 3DCP materials, the prototype dwelling has been designed to withstand local seismic activity and combines modern 3DCP techniques with traditional local craftsmanship in the form of a thatched roof. It showed a significant increase in ductility and energy absorption compared to conventional methods, proving the efficacy of 3DCP in seismic applications. The unnamed house was built using COBOD's BOD2 printer, which was also used on the recent post office in India and Europe's first two-story 3D-printed house.

These case studies highlight the enhanced performance of advanced materials over traditional solutions, demonstrating their potential to significantly reduce earthquake-related damage.
Challenges in Adoption
Despite the promise of these new materials, there are challenges to their widespread adoption. The cost of advanced materials like HPFRC and ECC is higher than traditional concrete, which can be a barrier in large-scale projects. Additionally, the availability of specialized materials and the equipment required for 3DCP can be limited in certain regions.
Moreover, these innovations demand specialized knowledge for proper application, meaning that contractors and engineers must undergo training to ensure correct usage. One notable example is the use of UHPC in the Perez Art Museum in downtown Miami. Built on Biscayne Bay, the museum faces challenges from sea air, salt exposure, and the risk of tropical storms and hurricanes.
UHPC was employed to produce 100 16-foot-long mullions, supporting the world’s largest impact-resistant window at the time (Fig. 9). This demonstrates the potential but also the complexity of using advanced materials in construction.
Fig. 9. Perez Art Museum, Downtown Miami Miami City,USA (Source: Miami New Times)
While construction with these materials can be costly at small scales, they offer long-term cost savings and environmental benefits (Table 2).
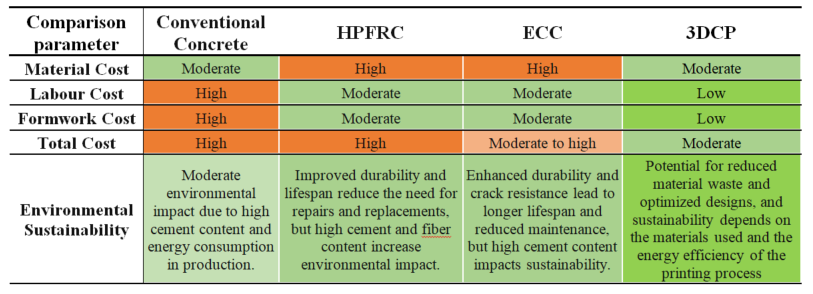
However, ongoing research and development are addressing these concerns, with efforts to lower production costs and improve material accessibility. As the industry becomes more familiar with these technologies, the integration of advanced materials into seismic-resistant designs is expected to become more commonplace.
Future Directions in Seismic-Resistant Materials
Looking forward, seismic-resistant materials are set to undergo even more groundbreaking advancements. Emerging smart materials (Fig. 10), capable of adapting in real-time to seismic forces, represent a cutting-edge solution. These materials are engineered to alter properties like stiffness, damping, flexibility and shape retention in response to external conditions, offering dynamic earthquake resistance. Examples of such innovative materials include:
Blue Mussels: Blue mussels anchor to rocks using adhesive byssal threads. Researchers are exploring how their flexibility can improve building resilience (Fig. 10a).
Shape Memory Alloys: Shape memory alloys represent a fascinating advancement in materials science, particularly for their application in earthquake-resistant construction (Fig. 10b).
BuildBlock ICF Blocks: This high-performance insulating concrete form (ICF) wall system consists of two 2 ½ inch Expanded Polystyrene (EPS) panels, connected by durable, high-density plastic webs, Its innovative design enhances structural integrity, making it ideal for earthquake-resistant construction (Fig. 10c).
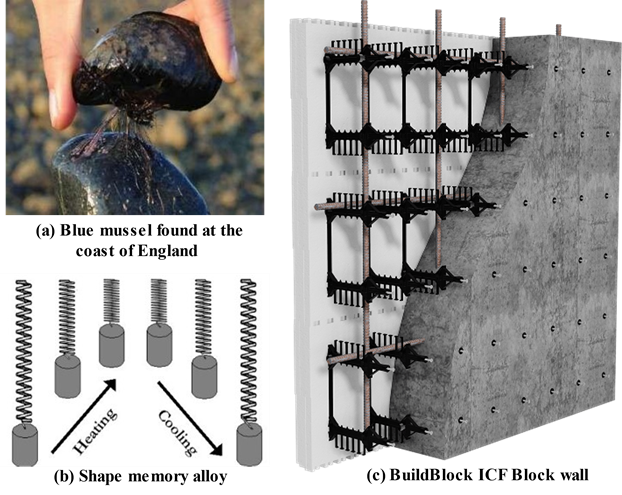
Structural resilience will be significantly increased by combining cutting-edge technologies to increase earthquake resistance with innovative materials, especially in harsh weather circumstances.
Below is a list of some examples of these developments (Fig. 11):
Levitating Houses: A Japanese system uses an airbag to lift a house 3cm during earthquakes, protecting it from damage (Fig. 11a).
Sou Fujimoto Architects: Sou Fujimoto Architects' floating tower design in Shenzhen's Qianhaiwan Bay showcases the future of earthquake-resistant structures with its innovative use of materials and resilient engineering (Fig. 11b).
Komatsu Seiren Fabric Laboratory Fa-Bo: An office building was renovated into the Fab Labo museum, using carbon fiber to enhance quake resistance. A regional rope braiding technique added flexibility to the carbon fiber (Fig. 11c).
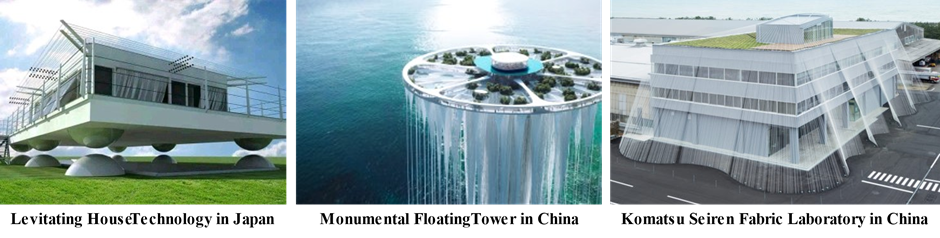
As research continues, the potential for innovative smart structures equipped with sensors and adaptive materials is becoming increasingly feasible. This could enable buildings to not only resist earthquakes more effectively but also communicate real-time data to engineers for maintenance and repair.
Conclusion
The adoption of innovative materials in seismic-resistant structures is a vital step toward ensuring the safety and resilience of infrastructure in earthquake-prone regions.
HPFRC, ECC, and 3DCP are transforming the way buildings are designed, offering superior ductility, energy absorption, and crack resistance. As the construction industry evolves, these materials are likely to become integral components of seismic design.
By overcoming the challenges of cost and specialized knowledge, and continuing to explore future advancements like smart materials, we can build more sustainable and earthquake-resilient cities. As seismic activity increases, the role of new materials in protecting lives and reducing structural damage becomes more critical than ever.
References:
Eurocode 8, design of structures for earthquake resistance. Part 1, General rules, seismic actions and rules for buildings. British Standards Institution, 2005.
Aashto, LRFD Seismic Bridge Design Published by the American Association of State Highway and Transportation Officials. 2011.
B. of Indian Standards, “IS 1893-1 (2002): Criteria for Earthquake Resistant Design of Structures, Part 1: General Provisions and Buildings.”
F. Gregor and Victor Li, “Influence-of-Matrix-Ductility-on-Tension-Stiffening-Behavior-of-Steel-Reinforced-Engineered-Cementitious-Composites-ECC,” Engineering, Materials Science, vol. 99, pp. 104–111, 2002, Accessed: Sep. 25, 2024. [Online]. Available: https://api.semanticscholar.org/CorpusID:137375199
S. Yi, N. Wilson, B. M. J, O. C. P, and B. S. L, “Seismic Performance of High-Performance Fiber-Reinforced Cement-Based Composite Structural Members: A Review,” Journal of Structural Engineering, vol. 148, no. 10, p. 03122004, Oct. 2022, doi: 10.1061/(ASCE)ST.1943-541X.0003428.
G. Jen, W. Trono, and C. P. Ostertag, “Self-consolidating hybrid fiber reinforced concrete: Development, properties and composite behavior,” Constr Build Mater, vol. 104, pp. 63–71, 2016, doi: https://doi.org/10.1016/j.conbuildmat.2015.12.062.
S. Bustan, F. Warsi, B. Panda, and P. Biswas, “Effect of strain hardening material on seismic performance of 3D printed concrete wall: a numerical study”, doi: 10.24355/dbbs.084-202408191226-0.
Z. Zhang and Q. Zhang, “Self-healing ability of Engineered Cementitious Composites (ECC) under different exposure environments,” Constr Build Mater, vol. 156, pp. 142–151, 2017, doi: https://doi.org/10.1016/j.conbuildmat.2017.08.166.
D. Zhang, P. Feng, P. Zhou, W. Xu, and G. Ma, “3D printed concrete walls reinforced with flexible FRP textile: Automatic construction, digital rebuilding, and seismic performance,” Eng Struct, vol. 291, p. 116488, 2023, doi: https://doi.org/10.1016/j.engstruct.2023.116488.